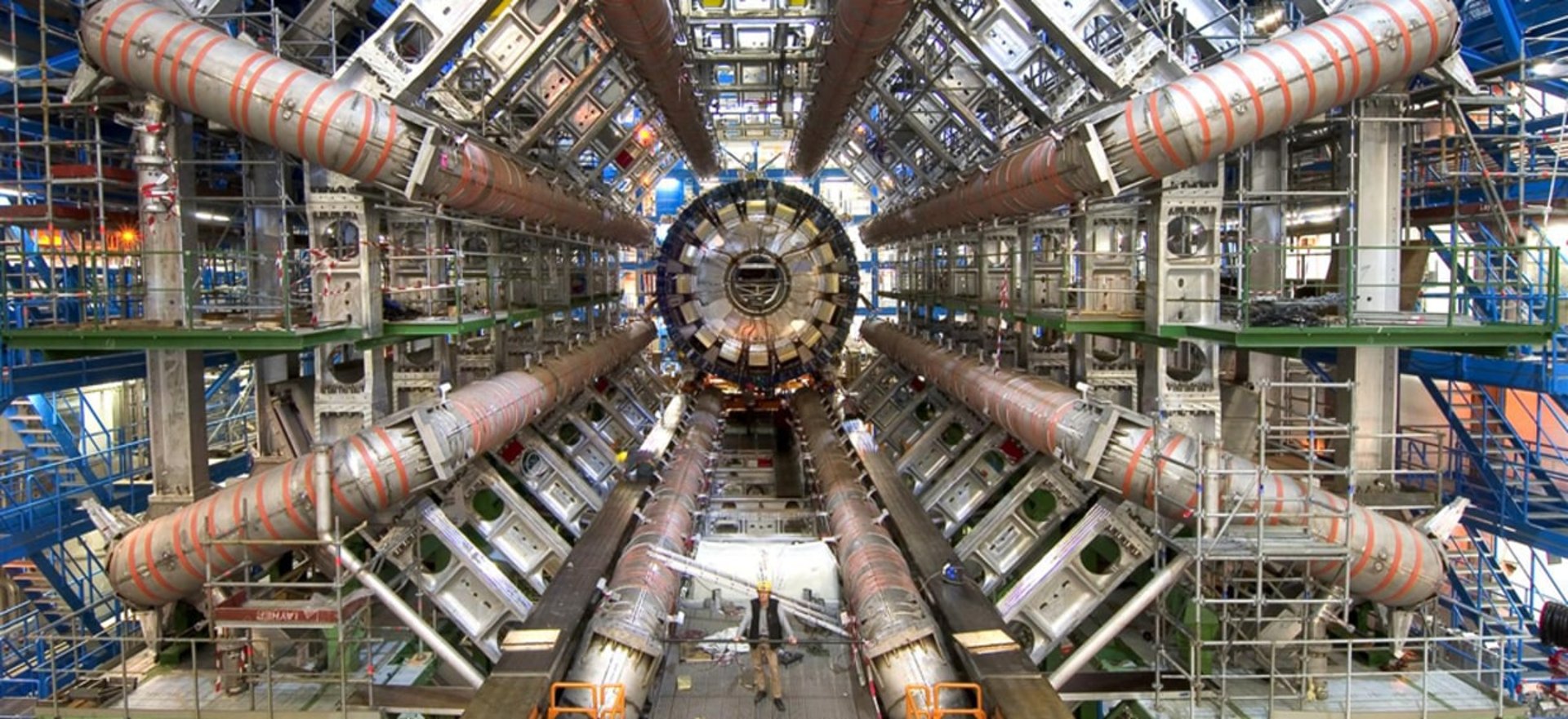
PHYSICS
Recent XENON1T Anomaly and its Implications
Dr. Ujjal Kumar Dey
Noble gases are so noble that not only they avoid reacting in mundane quotidian reactions but help us in search of dark matter (DM), the largest constituent form of matter present in our Universe. Decades of astrophysical observations ascertain the existence of DM. We postpone the discussion of chronological build-up of these evidences for some other time, here we just focus on the properties of DM that can be utilised to detect them directly and noble gases play a significant role here. From the observations it is known that DM does not interact with the electromagnetic field, and thus can not absorb, reflect or emit any type of electromagnetic radiation, and is therefore extremely difficult to detect. However, like any form of matter, DM must interact gravitationally. In fact till now the existence of DM can be inferred only from the gravitational effect it seems to have on normal matter. These indirect form of evidences include observations from galaxy rotations, collisions, formation and evolution of galaxies, gravitational lensing, cosmic microwave background etc. Now, any direct proof, or devising a direct search strategy for that matter, solely relies upon the exact nature of DM but that is still unknown. From the theoretical point of view, particle physics provides some interesting options. The primary candidate for DM is some new kind of, hitherto undiscovered, elementary particle. In many popular theories, e.g., supersymmetry, extra dimension etc., this class of particles are weakly interacting massive particles (WIMP).
The DM particles are chargeless, so in most cases it will not interact with the atomic electrons but will instead elastically scatter off the atomic nucleus. The resulting momentum transfer gives rise to a nuclear recoil which might be detectable. Based on this simple principle the paradigm of textit{direct detection} of DM is founded. Gravity-based observations of galaxies give us a fairly well-motivated density distribution and kinematics of DM particles in a galaxy. So we just have to build some kind of detectors on Earth and wait for some DM particle to pass through it and get detected. Here comes the ingenuity of noble gases to our rescue. Among all the noble gases, Ar and Xe are especially wellsuited for these purposes -- they are excellent scintillators (light pulses can be generated by the excited atom and detected, often with photo-multiplier tubes or PMTs) and can be ionized easily, their boiling points lie conveniently above the temperature of liquid nitrogen. Both of them can be liquefied to build dense and compact dark matter targets. This is why a number of direct detection experiments are devised using Ar and Xe.
One such ongoing experiment is the XENON dark matter experiment, installed underground at the Laboratory Nazionali del Gran Sasso of INFN, Italy. This is a 62 kg liquid Xe target operated as a dual phase (liquid/gas) time projection chamber (a type of particle detector that uses a combination of electric fields and magnetic fields to perform a three-dimensional reconstruction of a particle trajectory or interaction) to search for interactions of dark matter particles. See https://science.purdue.edu/xenon1t/?p=44, for a detailed account of the underlying detection principle. Actually an interaction in the target material generates scintillation light which is detected in the PMTs. There can be two types of recoils, namely, electronic and nuclear, and there are ways to distinguish between the two. The XENON collaboration recently reported the results of new physics searches with low-energy electronic recoil data obtained in the XENON1T detector [PRD 102, 072004]. They observed an excess at lower electron recoil energies 1-7 keV mostly crowded toward the lower energy ranges of 2-4 keV range, with 285 observed events as compared to 232$pm$15 events expected from known backgrounds. Neglecting a possible source of contamination from a tritium impurity in the detector the observation can be explained by the solar axions (a hypothetical elementary particle postulated to resolve the strong CP problem in quantum chromodynamics), or by assuming enhanced neutrino magnetic moments. In fact many other explanations are put forward soon after the observation was published.
Now, there can arise an interesting possibility. When a DM scatters with the nucleus of the detector atoms there is a time lag for the electron cloud to catch-up the recoiling nucleus, which results in a charge asymmetry. This can lead to an ionization and/or (de)excitation of the atoms, eventually contributing to the electron recoil signals in direct detection experiments. For light DM where the nuclear recoil is below threshold, these ionization (and sub-dominant bremsstrahlung process) signals provide a novel handle to search for them. This process of ionization or excitation of an atom is known as Migdal effect. For the n=3 and n=2 atomic states of the Xe atom, the ionization energies are 0.66 keV and 4.9 keV, respectively. This strikingly coincides with the generic energy scale of the observed XENON1T excess around 1-7 keV. Inspired by this in a recent paper [arXiv:2006.12529], we make a systematic study of a possible explanation of the observed excess events by considering the Migdal ionization effect with sub-threshold nuclear recoil. Remaining agnostic about the specific features of the DM particle and the underlying model thereof, we explore scenarios where the DM nucleus scattering cross-section is spin independent and spin-dependent as an explanation to the recently observed XENON1T excess. In future this proposal can be tested by the fact that the events generated from the excitation of the n=4 atomic levels electrons of the Xe atom may result in a peak at 61 eV. These may be readily explored with further lowering of the threshold of the direct detection experiments in the near future.