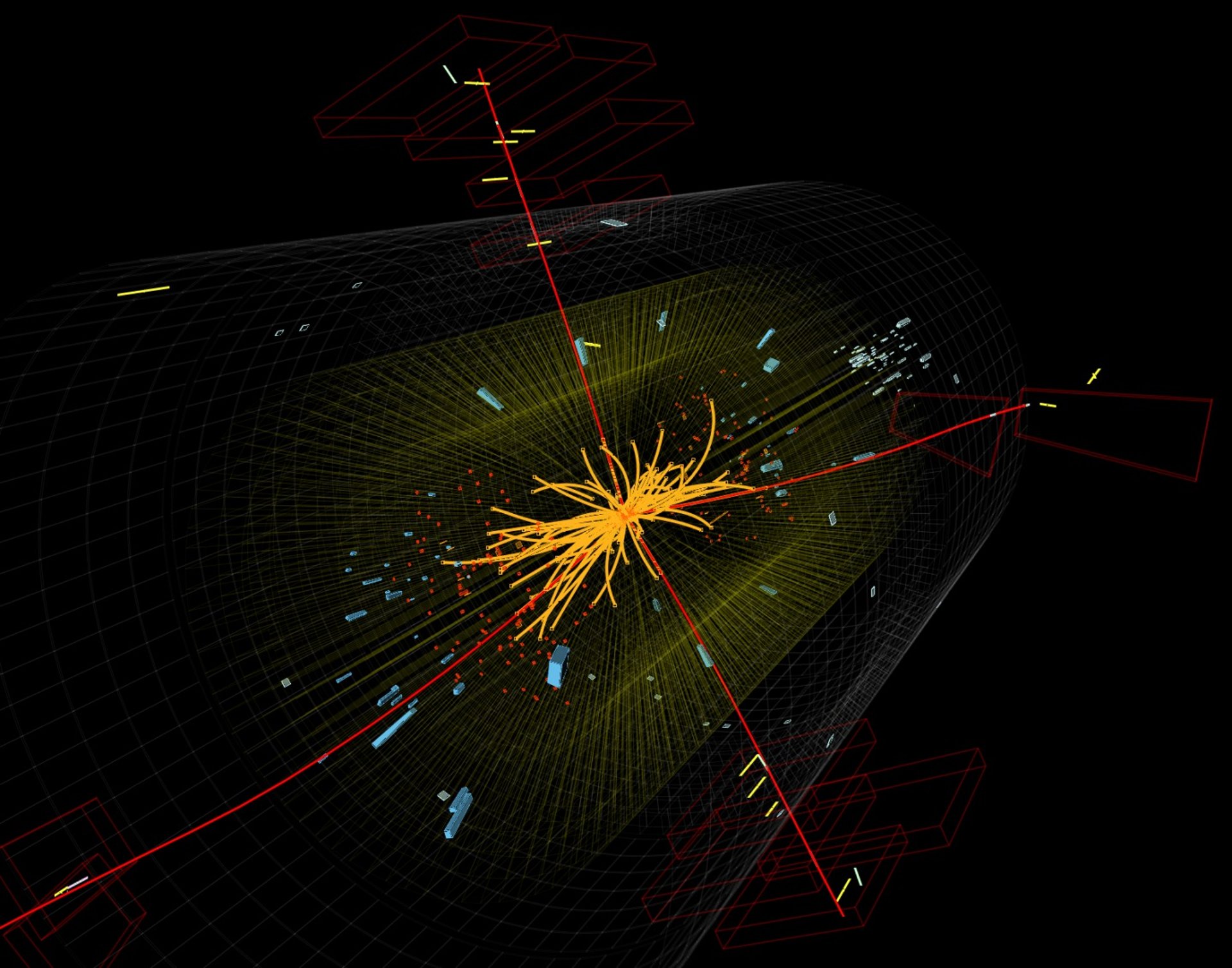
The Higgs Boson: A General Overview of its Discovery And Mass
Sourav Bera, 3rd Year BS-MS, Dept. of Physical Sciences, IISER Bpr
A round of ecstatic applause, whistles, and cheers filled the auditorium at CERN, near Geneva, Switzerland. Peter Higgs was himself present at the party. “Higgs boson-like particle discovery claimed at LHC”, the BBC news report said. On July 4, 2012, the ATLAS and CMS experiments at CERN’s Large Hadron Collider (LHC) announced that they had each observed a new particle in the mass region around 125 GeV. This particle was consistent with the long-sought Higgs Boson, the quest for which began in 1964. But what makes this particle so popular among physicists?
The Standard Model (SM) of particle physics is a complete theoretical framework that attempts to explain the properties of the fundamental constituents of matter and their interactions. The elementary particles are divided into three groups: Quarks (present inside the composite particles: neutrons and protons), Leptons (such as electrons, neutrinos), and Gauge Bosons, which are often called ‘force mediators’, some examples might be gluons as mediators of the strong force and photons as mediators of electromagnetic force. The SM implies that two of the four fundamental forces – the weak force and electromagnetic forces are unified i.e., electricity, magnetism, light, and some types of radioactivity are all manifestations of a single underlying force known as the electroweak force[1]. This unified theory describes the electroweak force and its associated force-carrying particles, namely the photon, and the W and Z bosons (mediators of the weak force), except for a major glitch[1]. Model (SM) has been developed on the principle of local Gauge invariance and massless gauge bosons are a natural consequence of such symmetry. An elaborate study of these requires rigorous group theoretical and mathematical calculations which I am neglecting here and just focusing on the facts. Now, what’s the problem with this Standard Model? Experimentally, it is found the W and Z bosons are massive, about 100 times heavier than the proton, which violates the aforesaid conclusion[1]. The electroweak symmetry breaking due to the Higgs field by Higgs mechanism as proposed by the 1964 PRL paper (“Broken Symmetries and Masses of Gauge Bosons”) by Peter Higgs solves this problem[2]. What we now call the Brout-Englert-Higgs mechanism, gives mass to the W and Z bosons when they interact with an invisible field, the Higgs field, which pervades the universe.[1] Thanks to this discovery the Noble Prize in Physics 2013 was given to Francois Englert and Peter Higgs with the motivation “for the theoretical discovery of a mechanism that contributes to our understanding of the origin of mass of subatomic particles.”[1] Now let’s discuss the Higgs field and its associated Higgs particle.
The Higgs field is a scalar field that is controlled by a Mexican-Hat-shaped potential. Just after the Big Bang, the Higgs field was zero, but as the universe cooled and the temperature fell below a critical value, the field grew spontaneously so that any particle interacting with it acquired a mass[1]. The more a particle interacts with this field, the heavier it is[1]. Particles like the photon that do not interact with it are left with no mass at all[1]. A very popular analogy could be cited here, imagine Margaret Thatcher making her way through a crowded room; most of the people in her vicinity would naturally veer towards her owing to her authority and influence. So the distribution of the crowd in the room – analogous to the Higgs field – would get distorted as more and more people have shifted closer to the Iron Lady, thereby providing more mass to her.” This metaphorically, is what happens when a particle moves through the Higgs field. This field may be excited, which oscillates, much like the ripples that appear on a disturbed pond, and like all other fundamental fields, we observe the excitation of the Higgs field as a Higgs particle[3]. So, the Higgs particle is the quantized manifestation of the Higgs field. Thus Higgs field provides mass to other particles and the particle associated, Higgs particle, is sometimes regarded as the ‘God particle', though many scientists don’t like such nomenclature.
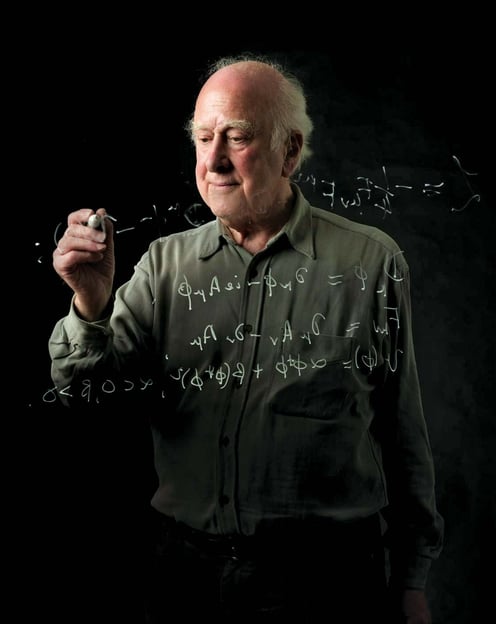
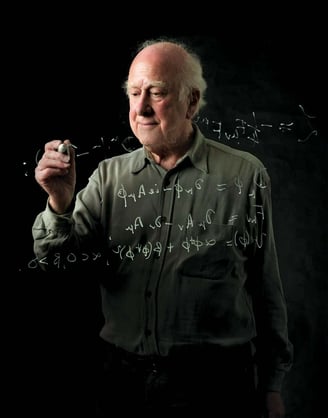
Peter Higgs was born in Newcastle upon Tyne in the UK, to a Scottish mother and an English father who worked as a sound engineer at the BBC. He received part of his early education at his home in Bristol before moving to London to study math and physics at age 17. He received his Ph.D. from King’s College in 1954. He then moved to the University of Edinburgh, where he has remained, with the exception of a few years spent in London in the late 1950s.
According to modern physics, matter consists of a set of particles that act as building blocks. Between these particles lie forces that are mediated by another set of particles. A fundamental property of the majority of particles is that they have a mass. Independently of one another, in 1964 both Peter Higgs and the team of François Englert and Robert Brout proposed a theory about the existence of a particle that explains why other particles have a mass. In 2012, two experiments conducted at the CERN laboratory confirmed the existence of the Higgs particle.
Source: Peter Higgs – Facts. NobelPrize.org. Nobel Media AB 2019. Sun. 14 Apr 2019.
The SM of particle physics describes Higgs particle (elementary particle) as boson of spin 0 and electric charge 0[4]. Though Higgs field provides mass to other particles, the mass of the Higgs particle is not known a priori and is a free parameter but is bounded by some upper and lower bounds owing to certain theoretical arguments. This particle is also not stable (lifetime of 1.6 ×10-22s as predicted by SM) [5], and it decays through different ways into lighter particles such as fundamental fermions like quarks or leptons, pairs of heavy bosons or pairs of massless bosons (photons or gluons)[6]. This process can follow a number of paths and each of these possible paths of decaying has its own probability of occurrence, but it’s not properly clear which path is favored over the other. To determine the mass of the Higgs boson, scientists usually compare the decay paths
they observed after a particle collision to the decay paths they simulated with computers and map out for a possible range of Higgs masses[7]. LHC discovered Higgs particle, by colliding two beams of protons each accelerated to an energy of nearly 3.5 TeV, huge energy getting converted to mass according to Einstein’s E = mc2, and observed a new particle in the mass region around 125 GeV that decayed into photons and Z bosons, consistent with the Higgs boson predicted by SM.[3] When the Higgs boson was discovered at the LHC, it was being produced about once per four billion proton-proton collisions![3]
The Higgs is the keystone of the SM and hence everything we learn about this new particle is central to the deepest laws of nature. If it does not exist, physicists will have to come up with a new model of how our universe works. Fortunately, the Higgs boson has been discovered but understanding its properties is still a big challenge. Studying those would further lead to the doorway beyond SM to the physics of Dark Matter, neutrino physics, theories with Supersymmetry and extra dimensions, etc. Recently, International Collaboration for a post - LHC Future Circular Collider (FCC) has been proposed for these purposes.
[1] https://home.cern/science/physics/higgs-boson
[2] P. W. Higgs (1964); “Broken Symmetries and Masses of Gauge Bosons”, Phys. Rev. Lett.,13,508.
[3] “The Higgs adventure: five years in CERN”: https://cerncourier.com/the-higgs-adventure-five-years-in/
[4] The ATLAS Collaboration; “Observation of a New Particle in the Search for the Standard Model Higgs Boson with the ATLAS Detector at the LHC”; Phys. Lett. B.,716(1): 1-29.
[5] Dittmaier, Mariotti, Passarino, Tanaka, Alekhin, Bagnaschi, Banfi (2012); “Handbook of LHC Cross sections: 2. Differential Distributions”. CERN Report 2(Tables A.1 – A.20). 12.01:3804.
[6] David d’Enterria (2012), “On the (Gaussian) maximum at a mass mH ≈ 125 GeV of the prod- uct of decay probabilities of the Standard Model Higgs boson”; arXiv: 1208.1993v2 [hep-ph].
[7] Fermilab article: http://news.fnal.gov/wp-content/uploads/higgs-boson.pdf
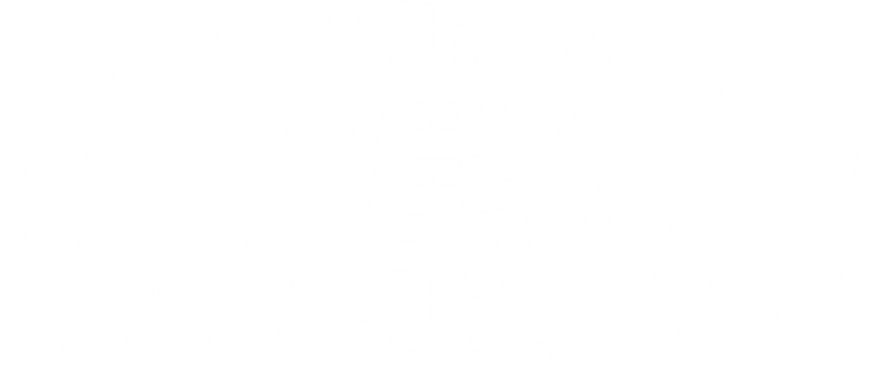
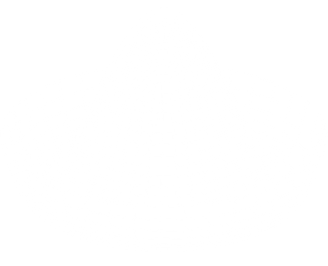